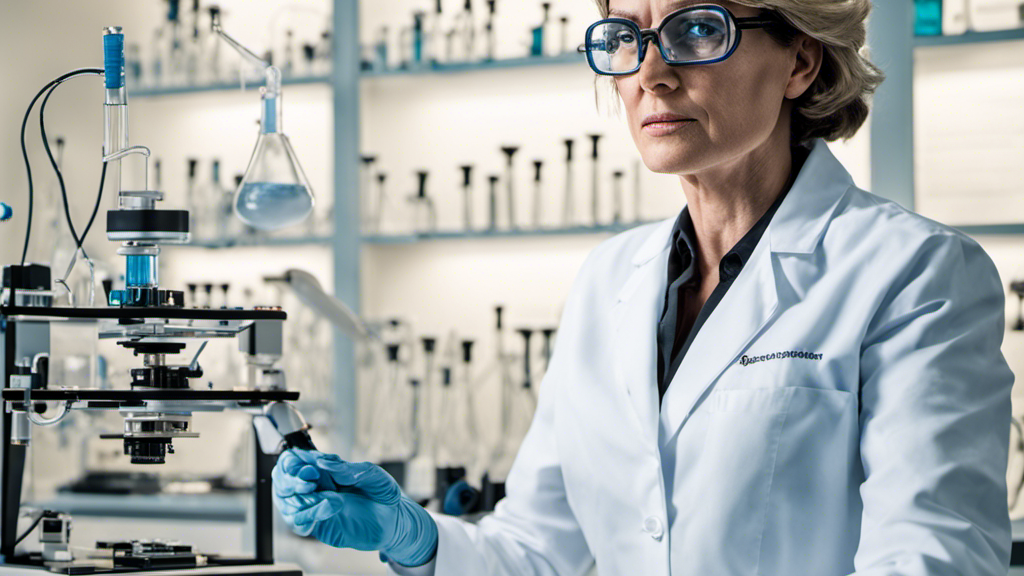
Latest Discoveries in Epigenetics
Discover the latest advancements in epigenetics, including algorithms, biomarkers, and feedback regulation. Explore the potential applications in medicine and genetics, and the implications for cancer treatment strategies.
1. Introduction to Epigenetics
Brief overview of epigenetics
Epigenetics is a rapidly growing field of research that focuses on changes in genetic information that do not involve alterations in the DNA sequence itself. Instead, these changes rely on mechanisms such as DNA methylation, histone modification, and chromatin remodeling, which can influence gene expression and regulation Muñoz-Cánoves and Di Croce, 2015. Epigenetic modifications play a crucial role in various biological processes, including cellular differentiation, development, and disease progression Riddihough and Zahn, 2010.
Importance of epigenetic research
The study of epigenetics has the potential to revolutionize our understanding, diagnosis, treatment, and prevention of various neurological and psychiatric disorders, as well as other diseases such as cancer Grezenko et al.. By unraveling the intricate interplay between genetic, epigenetic, environmental, and lifestyle factors, researchers can gain insights into the complex landscape of these conditions and develop personalized medicine, early intervention, and public health strategies.
For example, a considerable proportion of human malignancies, including ocular surface squamous neoplasia, are believed to be associated with global DNA hypomethylation and localized hypermethylation at promoters of certain genes Shahraki et al., 2023. Understanding the role of epigenetic mechanisms in these diseases can help identify novel therapeutic targets and improve patient care.
Recent advancements and discoveries
Recent discoveries in epigenetics have shed light on various aspects of gene regulation, chromatin structure, and cellular differentiation. Some of these advancements include the development of epigenetic algorithms for chromosome regulation, the study of phenotypic switching and cancer drug resistance, and the investigation of epigenetic feedback regulation and homeorhesis Muñoz-Cánoves and Di Croce, 2015.
Moreover, researchers are exploring epigenetics-driven chromosome folding, epigenetic biomarkers in blood samples, and the potential of epigenetic therapy for various diseases, including chordoma, a rare type of bone cancer Martinez Moreno et al., 2023.
As the field of epigenetics continues to expand, interdisciplinary efforts, ethical diligence, innovative technologies, and translating scientific insights into real-world applications will be crucial for unlocking its vast potential in improving human health.
2. Epigenetic Algorithms and Chromosome Regulation
Epigenetic Algorithms and Chromosome Regulation
Epigenetic mechanisms, such as DNA methylation, histone modification, and chromatin remodeling, play a crucial role in regulating gene expression and maintaining cellular functions. Recent advancements in epigenetic research have led to the development of novel algorithms and models to better understand the complex interactions between epigenetic modifications and chromosome regulation.
One such advancement is the Self-Reinforcement-Attention mechanism, which has been shown to impact gene expression and regulation. This mechanism is based on the concept of the imprintome, a specific subset of genes whose expression is controlled by epigenetic marks that can be modified by lifestyle factors, including diet, behavior, environment, and social interactions (Bland, 2021). The imprintome acts as a rapid translator for real-time changes, responding to environmental and lifestyle inputs by genomic methylation and histone modifications that affect promoter accessibility and transcription factor activity.
In a study investigating the stability of DNA methylation measurements over time and in the presence of acute stress, researchers found that varying time intervals, acute stress, and early life adversity (ELA) exposure influenced the stability of repeated DNA methylation measurements (Apsley et al., 2023). Interestingly, the study also revealed that probes used in most epigenetic-based algorithms for estimating epigenetic age or immune cell proportions had average or below-average stability, except for the Principal Component and DunedinPACE epigenetic aging clocks, which were enriched for more stable probes.
Another area of interest in epigenetic research is the role of testis-specific histone H2B variant (TH2B) in sperm function and embryo development. A recent study identified the genomic loci associated with TH2B in fertile human sperm by ChIP-seq analysis, revealing that approximately 5% of the sperm genome and 5527 genes were associated with TH2B (Patankar et al., 2021). The study found that TH2B enrichment was more prevalent in promoter and gene body regions, and its distribution varied across chromosomes. The altered expression of TH2B-associated genes in infertile individuals with sperm chromatin compaction defects indicates the involvement of TH2B in transcriptional regulation of these genes in post-meiotic male germ cells.
Epigenetic-mediated regulation of gene expression is also crucial for maintaining the fidelity of mechanisms governing the cell cycle. In a recent review, researchers discussed the complex and interdependent processes that control cell proliferation and are compromised in cancer, as well as the epigenetic and topological domains associated with distinct phases of the cell cycle that may be altered in cancer initiation and progression (El Dika et al., 2022). The review also highlighted the requirement for mitotic bookmarking to maintain intranuclear localization of transcriptional regulatory machinery to reinforce cell identity throughout the cell cycle and prevent malignant transformation.
These latest discoveries in epigenetic algorithms and chromosome regulation have the potential to significantly impact medicine and genetics by providing a deeper understanding of gene expression and regulation, as well as offering new avenues for therapeutic interventions in various diseases, including cancer.
3. Phenotypic Switching and Cancer Drug Resistance
Role of Epigenetics in Cancer Drug Resistance
Cancer drug resistance is a major challenge in cancer treatment, and while genetic mutations have been traditionally considered the primary cause, recent studies have highlighted the role of non-genetic mechanisms, such as epigenetic modifications, in the emergence of acquired drug resistance [Bhattacharya et al., 2021]. Epigenetic reprogramming, including DNA methylation and histone modification, can regulate the expression of genes involved in cellular proliferation, immune checkpoints, and the response to anti-cancer drugs [Yeon et al., 2020]. Understanding the role of epigenetics in cancer drug resistance can lead to more effective treatment strategies and potentially delay or minimize the emergence of resistance.
Phenotypic Switching Mechanisms
Phenotypic switching is a process by which cancer cells can acquire transient resistant phenotypes through non-genetic mechanisms, such as epigenetic modifications and stochastic fluctuations in gene expression [Gunnarsson et al., 2020]. Although these resistant phenotypes are eventually relinquished by individual cells, they can temporarily protect the tumor from extinction and enable the emergence of more permanent resistance mechanisms. Chronic stress after drug treatment can induce intrinsic cellular reprogramming and cancer stemness through a slow-cycling persister state, which subsequently drives cancer progression [Ravindran Menon et al., 2020]. Both epigenetic and metabolic mechanisms play an important role in this dynamic process.
Implications for Cancer Treatment Strategies
The understanding of phenotypic switching and the role of epigenetics in cancer drug resistance has generated interest in the potential of epigenetic therapies for long-term tumor control or eradication. Mathematical models have shown that even short-term epigenetic modifications and stochastic fluctuations in gene expression can drive long-term drug resistance in the absence of any bona fide resistance mechanisms [Gunnarsson et al., 2020]. Furthermore, an epigenetic drug that slightly perturbs the average retention of the resistant phenotype can turn guaranteed treatment failure into guaranteed success.
Combining an epigenetic drug with an anti-cancer agent can significantly outperform monotherapy, and treatment outcome is heavily affected by drug sequencing [Gunnarsson et al., 2020]. For example, histone deacetylase inhibitors (HDACs) have been shown to regulate the expression of immune checkpoint molecules, such as programmed death-1 (PD-1) and programmed death ligand-1 (PD-L1), and genes involved in immune evasion, making them ideal targets for the development of anti-melanoma therapeutics [Yeon et al., 2020]. By understanding the role of group behavior and the underlying non-genetic mechanisms in cancer drug resistance, researchers can develop more efficacious treatment designs that minimize or delay the emergence of resistance [Bhattacharya et al., 2021].
4. Epigenetic Feedback Regulation and Homeorhesis
Waddington’s Landscape and Epigenetic Feedback
Epigenetic feedback regulation plays a crucial role in cellular differentiation and development. Waddington’s landscape, a concept introduced by Conrad Waddington in the 1940s, describes the process of cellular differentiation as a ball rolling down a landscape with multiple valleys representing different cell fates. The landscape is shaped by the interplay between genetic and epigenetic factors that guide the cell towards a specific fate. Recent studies have highlighted the importance of epigenetic feedback regulation in shaping the Waddington’s landscape and determining cellular outcomes [Jia et al., 2019].
Role in Cellular Differentiation and Development
Epigenetic feedback regulation can influence the dynamics of cellular processes such as the epithelial-mesenchymal transition (EMT), which is critical in cancer metastasis and chemoresistance. EMT is regulated at multiple levels, including transcriptional, translational, protein stability, and epigenetics. The mechanisms by which epigenetic regulation can alter the dynamics of EMT remain elusive. However, incorporating a feedback term in the miR-200/ZEB/miR-34/SNAIL circuit, a model of EMT regulation, has been shown to stabilize the mesenchymal state and make transitions out of that state difficult [Jia et al., 2019]. This suggests that epigenetic feedback regulation can play a significant role in determining cellular outcomes and maintaining cellular homeostasis.
Potential Therapeutic Applications
Understanding the role of epigenetic feedback regulation in cellular differentiation and development has potential therapeutic applications in various diseases, including cancer. For example, targeting epigenetic feedback mechanisms that stabilize the mesenchymal state in EMT could help design more effective cancer therapeutics, as EMT is often associated with cancer metastasis and chemoresistance [Jia et al., 2019].
Moreover, epigenetic feedback regulation has been implicated in the metabolic response of growing German Simmental bulls fed rations low in crude protein and supplemented with rumen-protected methionine (RPMET). The addition of RPMET altered the hepatic anti-oxidant status in these bulls compared to both control groups, suggesting a role for epigenetic feedback regulation in nutrient partitioning and metabolic prioritization [Inhuber et al., 2023]. This finding could have implications for livestock management and the development of dietary strategies that optimize animal health and productivity.
In conclusion, epigenetic feedback regulation plays a critical role in shaping Waddington’s landscape and influencing cellular differentiation and development. Understanding the mechanisms underlying epigenetic feedback regulation could lead to the development of novel therapeutic strategies for various diseases, including cancer, and contribute to optimizing animal health and productivity.
5. Epigenetics-Driven Chromosome Folding
Epigenetics-Driven Chromosome Folding
The three-dimensional (3D) folding of chromosomes plays a crucial role in regulating gene expression and other genetic processes, such as transcription, DNA repair, and epigenetics. Recent advancements in chromosome capture methods, such as Hi-C, have allowed researchers to construct contact maps that depict 3D interactions among all DNA segment pairs, revealing a complex cross-scale organization spanning megabase-pair compartments to short-ranged DNA loops (Bernenko et al., 2023). To better understand the organizing principles behind chromosome folding, researchers have analyzed Hi-C data using various models and algorithms.
One recent study employed the generalized Louvain algorithm to extract 3D communities from Hi-C experiments, treating the measured DNA-DNA interactions as a weighted network (Bernenko et al., 2023). This approach allowed the researchers to seamlessly scan through the community size spectrum, from A/B compartments to topologically associated domains (TADs). By constructing a hierarchical tree connecting these communities, the study found that chromosomes exhibit a significant portion of nested and non-nested community pairs alongside considerable randomness. Furthermore, nested parts were often associated with active chromatin, highlighting the importance of cross-scale relationships in chromosome folding models (Bernenko et al., 2023).
Another study developed a high-throughput DNA or RNA labeling technique called HiDRO (High-throughput DNA or RNA labeling with optimized Oligopaints) to identify factors that influence genome folding during interphase (Park et al., 2023). By screening the human druggable genome, the researchers identified over 300 factors that impact chromosome folding. Among these, 43 genes were validated as either increasing or decreasing interactions between TADs. The study also demonstrated the importance of GSK3A signaling in nuclear architecture and the use of HiDRO for identifying mechanisms of spatial genome organization (Park et al., 2023).
Single-molecule experiments have also provided insights into the structural composition and mechanical properties of structural maintenance of chromosome (SMC) proteins, which play a crucial role in DNA organization (Freitag et al., 2022). One study used optical tweezers unfolding experiments to investigate the isolated Psm3 coiled-coil (CC) as a model system. The researchers found that the CC elbow, a flexible and potentially non-structured feature, divides the CC into sections and induces a pairing shift from one CC strand to the other. This finding suggests that the CC elbow could facilitate large-scale conformational changes, most likely via thermal fluctuations of the flanking CC sections (Freitag et al., 2022).
Polymer physics models have also been employed to understand the mechanisms underlying the complex 3D architecture of mammalian genomes (Conte et al., 2023). Two major physical processes of chromatin organization, loop-extrusion and polymer phase-separation, have been implemented into these models. By testing these models against available single-cell super-resolution imaging data, researchers have shown that both mechanisms can cooperate to shape chromatin structure at the single-molecule level. Furthermore, these polymer models can be used as powerful tools to make predictions in silico that complement experiments in understanding genome folding, such as predicting chromatin structure rearrangements upon disease-associated mutations and identifying putative chromatin organizing factors that orchestrate the specificity of DNA regulatory contacts genome-wide (Conte et al., 2023).
In summary, recent discoveries in epigenetics-driven chromosome folding have shed light on the complex organization and regulation of 3D chromatin structures. By leveraging advanced techniques and models, researchers are gaining a deeper understanding of the mechanisms behind chromosome folding and their implications for gene expression and regulation. These insights hold great potential for future applications in medicine and genetics, including the development of novel therapeutic strategies and diagnostic tools.
6. Epigenetic Biomarkers in Blood Samples
Epigenetic Biomarkers in Blood Samples
Epigenetic biomarkers have emerged as a promising tool for the diagnosis and treatment of various diseases, including cancer. These biomarkers can be identified in blood samples, providing a non-invasive method for detecting epigenetic changes associated with disease progression and response to treatment. Recent studies have focused on the identification of pan-cancer epigenetic biomarkers and the use of statistical analysis software (SAS) to aid in their identification.
Pan-Cancer Epigenetic Biomarker Selection
Epigenetic alterations, such as DNA methylation and histone modifications, have been shown to play a significant role in the response to various anticancer treatments, including DNA damaging agents like topoisomerase I (Top I) inhibitors (Madkour et al., 2023). Identifying epigenetic biomarkers that can predict the response to these treatments is crucial for improving their efficacy and safety. In addition to cancer, epigenetic biomarkers have also been studied in other diseases, such as gestational diabetes mellitus (Linares-Pineda et al., 2023) and Parkinson’s disease (Tsalenchuk et al., 2023).
Use of SAS for Biomarker Identification
Statistical analysis software (SAS) has been employed to identify differentially methylated positions (DMPs) in blood samples, which can serve as potential epigenetic biomarkers for various diseases. For example, in a study of gestational diabetes mellitus, researchers used SAS to analyze DNA methylation patterns in peripheral blood samples from pregnant women, identifying over 1,000 DMPs that were significantly associated with the disease (Linares-Pineda et al., 2023). These DMPs were then correlated with biochemical variables such as glucose levels, cholesterol, and HbA1c, providing insights into the potential metabolic pathways related to gestational diabetes.
Potential Applications in Cancer Diagnosis and Treatment
The identification of epigenetic biomarkers in blood samples has significant implications for cancer diagnosis and treatment. For instance, the combination of Top I inhibitors with inhibitors of histone-modifying enzymes has been shown to enhance cytotoxic effects and sensitize resistant cells to Top I inhibitors (Madkour et al., 2023). Additionally, microRNAs and long non-coding RNAs (lncRNAs) have been found to influence the expression of Top I and other proteins in cancer cells, resulting in positive or negative alterations in the response to Top I inhibitors.
Moreover, epigenetic biomarkers have been studied in the context of type 2 diabetes mellitus (T2DM), with findings suggesting that epigenetic changes are involved in the disease’s risks, pathogenesis, and complications, as well as the development of new therapeutic targets (Mannar et al., 2023). In the case of lean nonalcoholic fatty liver disease (NAFLD), epigenetic mechanisms such as DNA methylation, histone modifications, and noncoding-RNA-mediated gene regulation have been implicated in the disease’s regulation, offering potential therapeutic options and noninvasive biomarkers (Aggeletopoulou et al., 2023).
In conclusion, the identification of epigenetic biomarkers in blood samples holds great promise for improving the diagnosis and treatment of various diseases, including cancer and metabolic disorders. The use of statistical analysis software for biomarker identification, coupled with a growing understanding of the role of epigenetic mechanisms in disease pathogenesis, offers new opportunities for the development of targeted therapies and noninvasive diagnostic tools.
7. Conclusion
Conclusion
The field of epigenetics has witnessed significant advancements and discoveries in recent years, contributing to our understanding of gene expression, regulation, and the potential for therapeutic interventions. These latest discoveries in epigenetics have shed light on various aspects of gene regulation, such as the Self-Reinforcement-Attention mechanism in chromosome regulation, phenotypic switching in cancer drug resistance, and epigenetic feedback regulation in homeorhesis, among others [1].
One of the most promising areas of epigenetic research is the development of epigenetic biomarkers for cancer diagnosis and treatment. The identification of pan-cancer epigenetic biomarkers in blood samples has the potential to revolutionize cancer diagnosis and treatment strategies [2]. Furthermore, understanding the role of epigenetic modifications in cancer drug resistance can lead to the development of novel therapeutic approaches to overcome this challenge [3].
Epigenetic research also extends beyond human health, with advances in crop biotechnology highlighting the potential for epigenetic modifications to improve agricultural practices and food security [4]. The growing body of knowledge in epigenetics has the potential to impact various fields, from medicine to agriculture.
Despite these promising advancements, challenges remain in fully understanding the complex mechanisms underlying epigenetic regulation and their implications for human health and disease. Future research should focus on elucidating the intricate relationships between epigenetic modifications and gene expression, as well as exploring the potential for epigenetic therapies in the treatment of various diseases. Additionally, the development of novel diagnostic and prognostic biomarkers based on epigenetic alterations can significantly improve patient outcomes and inform personalized treatment strategies.
In summary, the latest discoveries in epigenetics have significantly advanced our understanding of gene regulation and the potential for therapeutic interventions. As the field continues to grow, the opportunities for novel applications in medicine, agriculture, and other areas of research are vast. By addressing the challenges and harnessing the potential of epigenetic research, we can unlock new avenues for improving human health and well-being.
References
1. Muñoz-Cánoves P, Di Croce L. Special issue: epigenetics: introduction. 2015. https://europepmc.org/article/MED/25828932
2. Riddihough G, Zahn LM. Epigenetics. What is epigenetics? Introduction. 2010. https://europepmc.org/article/MED/21030643
3. Grezenko H, Ekhator C, Nwabugwu N, Ganga H, Affaf M, Abdelaziz A, Rehman A, Shehryar A, Abbasi F, Bellegarde S, Khaliq A. Epigenetics in Neurological and Psychiatric Disorders: A Comprehensive Review of Current Understanding and Future Perspectives. https://europepmc.org/article/PMC/PMC10446850
4. Shahraki K, Shahraki K, Ghasemi Boroumand P, Sheervalilou R. Promotor methylation in ocular surface squamous neoplasia development: epigenetics implications in molecular diagnosis. 2023. https://europepmc.org/article/MED/37493058
5. Martinez Moreno M, Wang E, Schroeder C, Sullivan P, Gokaslan Z. Shedding light on emerging therapeutic targets for chordoma. 2023. https://europepmc.org/article/MED/37647357
6. Apsley AT, Ye Q, Etzel L, Wolf S, Hastings WJ, Mattern BC, Siegel SR, Shalev I. Biological stability of DNA methylation measurements over varying intervals of time and in the presence of acute stress. 2023. https://europepmc.org/article/MED/37393564
7. Bland JS. A Discovery that Reframes the Whole of Global Healthcare in the 21st Century: The Importance of the Imprintome. 2021. https://europepmc.org/article/MED/34602872
8. Bao Y, Gorrie-Stone T, Hannon E, Hughes A, Andrayas A, Neilson G, Burrage J, Mill J, Schalkwyk L, Kumari M. Social mobility across the lifecourse and DNA methylation age acceleration in adults in the UK. 2022. https://europepmc.org/article/MED/36566336
9. Patankar A, Gajbhiye R, Surve S, Parte P. Epigenetic landscape of testis specific histone H2B variant and its influence on sperm function. 2021. https://europepmc.org/article/MED/33933143
10. El Dika M, Fritz AJ, Toor RH, Rodriguez PD, Foley SJ, Ullah R, Nie D, Banerjee B, Lohese D, Tracy KM, Glass KC, Frietze S, Ghule PN, Heath JL, Imbalzano AN, van Wijnen A, Gordon J, Lian JB, Stein JL, Stein GS. Epigenetic-Mediated Regulation of Gene Expression for Biological Control and Cancer: Fidelity of Mechanisms Governing the Cell Cycle. 2022. https://europepmc.org/article/MED/36348115
11. Yeon M, Kim Y, Jung HS, Jeoung D. Histone Deacetylase Inhibitors to Overcome Resistance to Targeted and Immuno Therapy in Metastatic Melanoma. 2020. https://europepmc.org/article/MED/32626712
12. Bhattacharya S, Mohant